- March 3, 2022
- Posted by: Stef Lantin
- Category: Research Spotlight
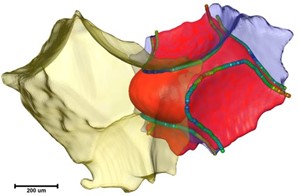
Ghent University’s Pore-scale Processes in Geomaterials Research group (PProGRess) studies the various physical, chemical and biological processes that occur inside porous geomaterials. Our group belongs to the department of Geology and specializes in non-destructive imaging of the 3D structure of porous materials, from nano- to macro-scale. As part of the Ghent University Centre for X-Ray Tomography (UGCT), we are closely involved in methodological work on improving real-time micro-CT imaging, in terms of both temporal and spatial resolution as its application to challenging in-situ experiments.
PProGRess evolved from the “Sedimentary Geology and Engineering Geology” research group, and therefore still holds a lot of expertise in the field of building materials (e.g. natural stone) and the assessment of their properties. Over the last few years, the application area of our research has expanded to include contaminant hydrogeology, subsurface energy storage, geological carbon storage, mineral (ore) characterization, industrial porous media and more. The group is led by Prof. Veerle Cnudde, and currently consists of 20 members, including 12 PhD students.
Research
PProGRess is active on a wide range of research subjects, commonly with the unifying characteristics that they involve processes in materials with a complex (micro-)structure, and that they benefit from advanced imaging methods. A few of our most recent topics are listed below.
Flow and transport in porous media
Understanding how fluids occupy and migrate through porous media is of great importance in hydrology and environmental engineering. PProGRess studies solute transport and colloid transport through porous media at the pore scale, in order to come to better models for contaminant transport in groundwater resources. Aside from giving information on how the pollutants themselves move, these methods also give crucial information on the underlying structure of the flow field, resulting in 3D micro-particle velocimetry (Fig. 1).
Figure 1: Tracks of colloidal micro-particles, suspended in a liquid flowing through the pores of a sandpack (left), as measured with fast laboratory-based micro-CT. This new 3D micro-particle velocimetry approach is one of the very few methods that can measure the 3D flow velocity field (right) inside an optically opaque porous material. Reference: Bultreys et al. 2022.
Another important subject is the simultaneous flow of multiple fluids in porous media (“multi-phase flow”, e.g. water/CO2 in subsurface CO2 sequestration or water/non-aqueous phase liquid in environmental engineering). Multi-phase flow in porous geo-materials is influenced by the connectivity and geometry of the pore space, as well as by solid/fluid/fluid interactions. PProGRess was one of the first groups to use fast micro-CT imaging to study fluid distributions and meniscus dynamics, particularly making the step from synchrotron to lab-based fast imaging (Fig. 2). We are also active in studying how well simplified models (e.g. pore network models) can capture the pore-scale physics at play. These studies are aimed to transform our understanding of wettability in porous media, and how it affects the resulting macro-scale behavior.
Contact angles: Curvatures (1/µm):
Figure 2: A fluid meniscus (red) halted in a pore throat between two pores (yellow and purple) of a bead pack, imaged with micro-CT. The figure on the left shows an image-based measurement of contact angles; the figure on the right shows image-based measurement of curvature, which indicates the pressure difference between the two fluids. Reference: Mascini et al. 2020.
References:
- Van Offenwert, S., Cnudde, V., & Bultreys, T. (2019). Pore-Scale Visualization and Quantification of Transient Solute Transport Using Fast Microcomputed Tomography. Water Resources Research, 55(11). https://doi.org/10.1029/2019WR025880
- Mascini, A., Boone, M., Van Offenwert, S., Wang, S., Cnudde, V., & Bultreys, T. (2021). Fluid Invasion Dynamics in Porous Media With Complex Wettability and Connectivity. Geophysical Research Letters, 48(22). https://doi.org/10.1029/2021GL095185
- Bultreys, T., Van Offenwert, S., Goethals, W., Boone, M. N., Aelterman, J., & Cnudde, V. (2022). X-ray Tomographic Micro-Particle Velocimetry in Porous Media. https://doi.org/10.48550/arXiv.2202.04114
Ice and salt crystallization in porous materials
Some of the main causes for building material degradation are weathering processes related to stresses induced by salt and ice crystallization in their pores. Determining these mechanisms is essential to produce better assessments of damage prevention and of the conservation of cultural heritage. Salt crystallization is also important in a different application area: storing renewable energy to balance out supply and demand. Geological structures, such as saline aquifers and depleted hydrocarbon reservoirs, possess large volumes of available pore space that can be used to store energy in the form of compressed air or hydrogen gas. However, these operations require the injection of gasses into pores that are typically filled with highly saline brine. Then, a part of the brine originally present inside the rock can evaporate, which can lead to salt precipitation. This can cause blockage in pores and throats, reducing the gas permeability, potentially leading to critical damage proximal to the injection well.
To understand and predict the influence salt and ice crystallization (Fig. 3) have on porous materials, it is important to understand the driving processes at the pore scale, which depends on the coupling between moisture migration, crystallization kinetics and micro-mechanics. We use fast micro-CT to study this, in controlled pressure, temperature and (moisture) flow conditions.
Figure 3: 3D renderings of an 87% water-saturated limestone sample in frozen (−10 °C) and thawed (10 °C) condition. The upper half of the sample is made invisible so that volumes of disappeared (red) and appeared mass (green) are clear. The damage resulting from the thawing depends on the water saturation. Source: Deprez et al. 2020.
References:
- Deprez, M., De Kock, T., De Schutter, G. and Cnudde, V. (2020). The role of ink-bottle pores in freeze-thaw damage of oolithic limestone. Construction and Building Materials, 246, 118515. https://doi.org/10.1016/j.conbuildmat.2020.118515.
- Derluyn, H., Boone, M., Desarnaud, J., Grementieri, L., Molari, L., de Miranda, S., Shahidzadeh, N., Cnudde, V. (2016). Quantifying salt crystallization dynamics in sandstone using 4D laboratory X-ray micro-ct. Science and Art: A Future for Stone, 83-90, https://biblio.ugent.be/publication/8518628.
Microbial colonization of porous sedimentary rocks
Bacteria have the potential to colonize rocks in natural outcrops and building stones. This can have both negative (i.e. biodeterioration) and beneficial effects (e.g. healing material defects through bio-cementation). The colonization potential (or bioreceptivity) is mainly related to the stone’s chemical composition, texture and structure and to the local environment. As bacteria colonize a stone, they can alter the stone’s properties through their metabolism (e.g. mineral precipitation and gas production) and through the formation of biofilms. We are interested in the interaction of the location, growth and activity of the biofilms and the petrophysical properties of rocks at the pore scale and vice versa. Studying this with micro-CT (Fig. 4) and SEM imaging allows us to find the precise mechanisms, the role of the organisms as well as the link between microbial colonization and petrophysical properties inside sedimentary rocks.
Figure 4: Cross-section through cyanobacterial biofilm covering Bentheim sandstone (8 mm x 7 mm x 16 mm). Source: PhD Thesis Laurenz Schröer.
Combining structural and chemical characterization of ore deposits
In the transition towards a circular economy, mineral resources need to be looked after carefully. The characterization of primary and secondary raw materials plays an important role in their recuperation and extraction. It is therefore necessary to couple microstructural and chemical information of these materials. The ore characterization field conventionally relies on hand specimens and thin/polished sections. Microscopic observations are therefore often limited to two-dimensional (2D) techniques (e.g. optical microscopy and SEM-EDS). These techniques are not capable of reproducing the real three-dimensional (3D) interior of geological samples. Conventional 3D imaging techniques such as micro-CT, on the other hand, offer limited chemical information. At PProGRess, we explore and implement non-destructive X-ray imaging techniques for the combined 3D chemical and structural characterization of ore deposits. We use novel lab-based hyperspectral X-ray micro-CT techniques for this (Fig. 5).
Figure 5: (a) and (b) reconstructed spectral micro-CT images of two different cross-sections through a natural rock sample (c) energy spectra of different particles indicated in (a and b) with the corresponding colors and theoretical positions of the Pb and the Au K-edges; note that the three spectra (red, yellow, and pink) possibly represented by sulfide minerals or silicates show no K-edge in the spectral range (NIST X-Ray Transition Energies Database; online available at:
physics.nist.gov/PhysRefData/XrayTrans/Html/search.html. Source: Sittner et al. 2020
References:
- Sittner, J., Godinho, J.R., Renno, A.D., Cnudde, V., Boone, M., De Schryver, T., Van Loo, D., Merkulova, M., Roine, A. and Liipo, J. (2020). Spectral X‐ray computed micro tomography: 3‐dimensional chemical imaging. X‐Ray Spectrometry, 50(2). https://doi.org/10.1002/xrs.3200.